For more than 20 years, SWEPOS has consistently demonstrated the full potential of real-time GNSS networks
Standing in the main control center of Sweden’s real time GNSS network (RTN) system, I feel like I could be standing in NASA Mission Control. A marvel of infrastructure created by Lantmäteriet, the country’s mapping agency, the center has huge screens displaying aspects of the SWEPOS RTN in real-time: CORS station health, monitoring station precision, GNSS systems status, server status, and caster activity. Staff members are monitoring the network to provide support for users. It’s no wonder that some of us RTN operators, who don’t have such resources, joke that we have a bit of “GNSS envy.”
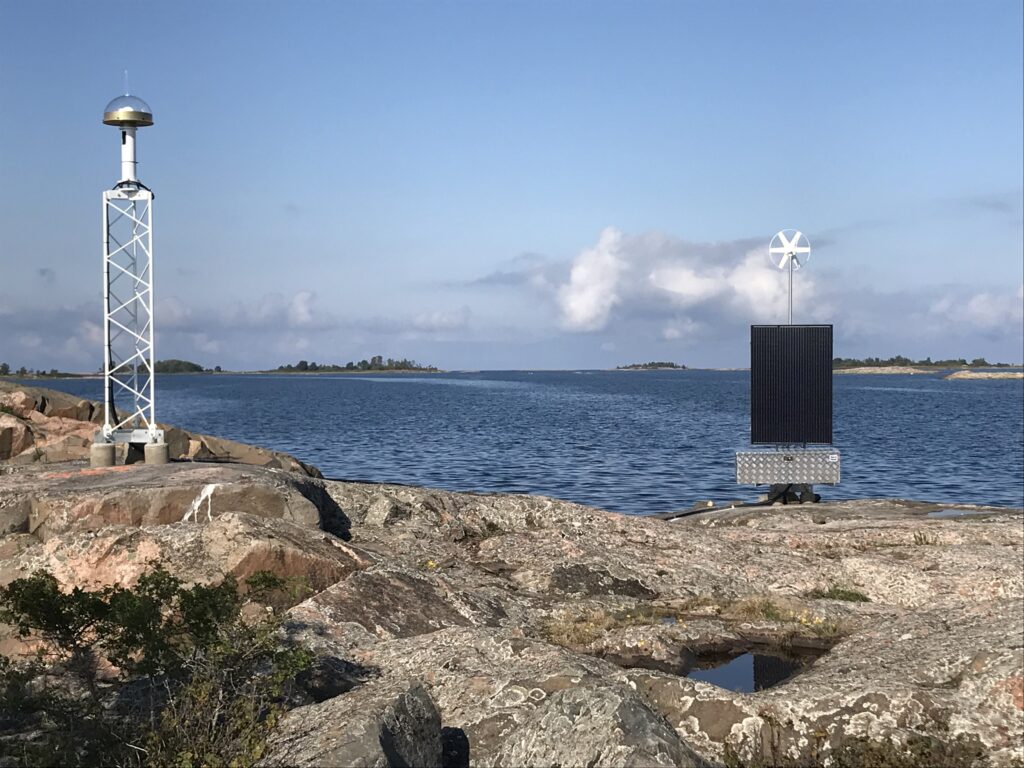
A continuously operating GNSS reference station on an islet off the east coast of Uppland Sweden. Many stations in the SWEPOS national GNSS network are co-located with other scientific, navigation, or meteorological sensors.
As a veteran RTN operator, I have followed the development of SWEPOS, the national RTN of Sweden, for many years and have been impressed with its progressiveness and innovation and have had the opportunity to talk with some of its notable innovators as well as witness first-hand how integral SWEPOS is to Sweden.
It is estimated that there are more than 500 RTN of various sizes and configurations operating worldwide. SWEPOS was not only an early adopter, it stands out as one of the best, checking all the boxes for what an RTN can deliver. In part, this is a function of how the RTN fits into the geospatial fabric of Sweden, especially for cadastral (real property) management.
Geospatial Modernization
Like many countries in the 1990s, Sweden was in the midst of modernizing its national positioning infrastructure. This included geodetic references: horizontal and vertical control (i.e. physical survey control marks), and other elements of physical geodesy, like gravity models. The recent advent of high-precision satellite positioning fundamentally changed the approach taken for this modernization in both a transition from analog to digital, and from “passive” to “active” control.
The primary shortcoming of legacy “passive” (i.e. physical) control monuments is their impermanence. In contrast “active” control is updated constantly. There was a global movement among the respective geodetic communities of many countries to develop networks of active control monuments in the form of continuously operating reference stations (CORS). These GNSS reference stations provide high-precision positions continuously.
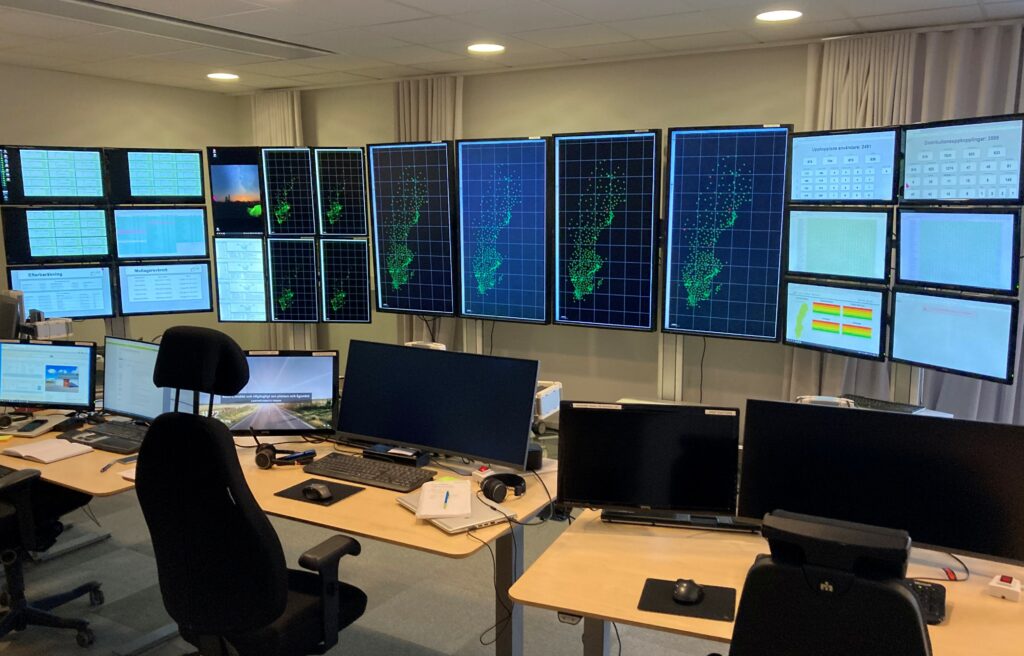
The main control and support center for SWEPOS, the national real-time GNSS network (RTN), located at the Lantmäteriet facility in Gävle Sweden.
This is in contrast to passive control marks, where the reference position could be potentially obsolete “as soon you walk away from it” (as the old saying goes among surveyors). A mark’s position can change due to plate tectonic shifts and other geophysical dynamics, subsidence, glacial rebound, and environmental factors, and can be damaged or destroyed by construction activities. CORS add an active temporal component, transforming 3D “XYZ” positions to 4D.
The geodetic and scientific value of CORS networks was well known, but it was also recognized that there would be many downstream benefits for surveying, construction, precision navigation, agriculture, mapping, public safety, and more—especially if the CORS network could also provide real-time GPS corrections.
As with many early RTN initiatives, Sweden’s began with a focus on surveying and geodetic applications.
“Some applications we looked at early on, like aerial survey using reference station data, showed many advantages,” says Peter Wiklund, section manager for SWEPOS within Lantmäteriet, the national property agency based in Gävle.
There was an opportunity to explore CORS and RTN development in cooperation with a prominent scientific institution, the Onsala Space Observatory, the Swedish National Facility for Radio Astronomy that is affiliated with the university in Gothenburg.
“Onsala had begun some tests with permanent reference stations,” says Wiklund. “Our first 20 stations were built in collaboration with Onsala with funding they secured. They had a background in satellite positioning, hosting a Doppler station from the days prior to GPS.”
This cooperation began in 1992 to explore building a CORS network, but also in anticipation of eventually providing real-time services. By the mid-1990s, real-time kinematics (i.e., using a base and rover) was developed, and by the end of the decade, so was Network RTK (aka NRTK or RTN). The advent of Network RTK would be the key driver for Lantmäteriet to build and host the SWEPOS RTN.
Land Matters
Lantmäteriet was a logical entity to develop and operate an RTN, as its functions would benefit substantially from real-time positioning. In addition to mapping the country and its properties, the agency provides services for subdivisions or changes in land boundaries, and handles applications for registered ownerships, ensuring that ownership registration is done in the real property register.
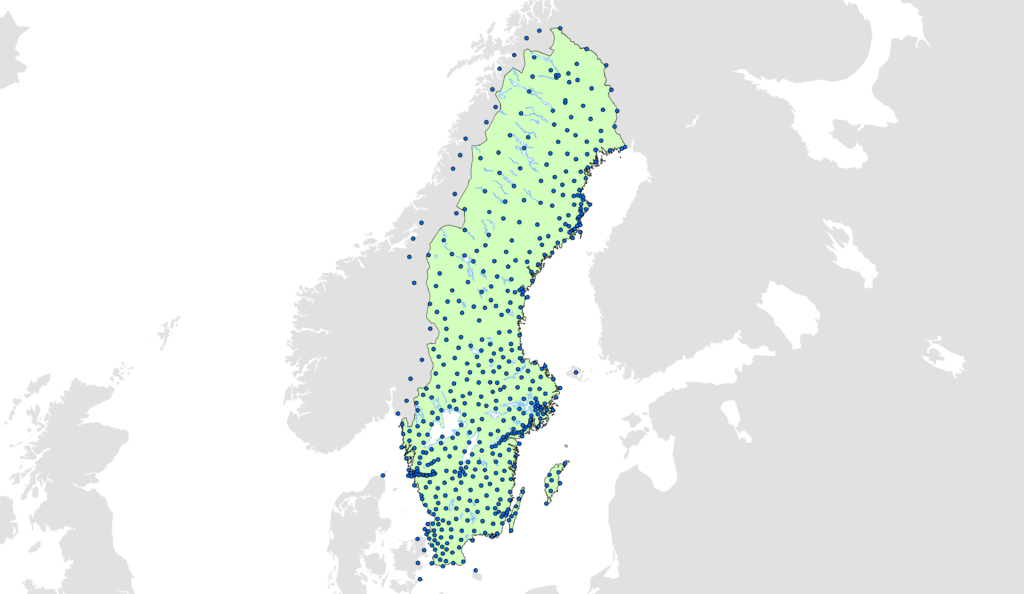
A map of the SWEPOS network of 470 continuously operating reference stations (CORS). —SWEPOS continues to densify, seeking a 35km nominal spacing—the densest large-scale RTN in the world.
In Sweden, and several other countries, all real-property surveys are performed by government authorities. Wiklund explains: “There are private surveyors for construction etc., but not within cadastral surveying. Any property survey is done by a government surveyor. It’s done by sovereign municipalities or the government. We are the government, and we are overseeing the cadastral operations of the municipalities.
“The cadastre defines all the houses in Sweden for real property,” he continues. “We hold this all in a large cadastral database that is also referenced for mortgages. We handle a lot of responsibility.”
A system like SWEPOS would enable Sweden to leverage a modernized reference framework for high precision and fidelity, as well as provide real-time efficiency.
The Network
SWEPOS encompasses 470 CORS. “Of those, 53 are Class A and very stable, mounted in bedrock for instance, while the other Class B CORS might be on buildings,” says Wiklund.
Lantmäteriet performs daily position calculations for its entire network. This is to look for movement of buildings, velocity from plate tectonics, and the significant effect of glacial rebound in many parts of Sweden. Most of the CORS are in Sweden, but there are several in adjacent countries to provide overlap for full coverage.
Nominal spacing of CORS for many RTN is 50km-70km. Lantmäteriet has done a lot of testing over the years and now strives for 35-km spacing to provide optimal results for its own needs and those of partners and customers. This makes SWEPOS the densest large-scale, real-time network in the world.
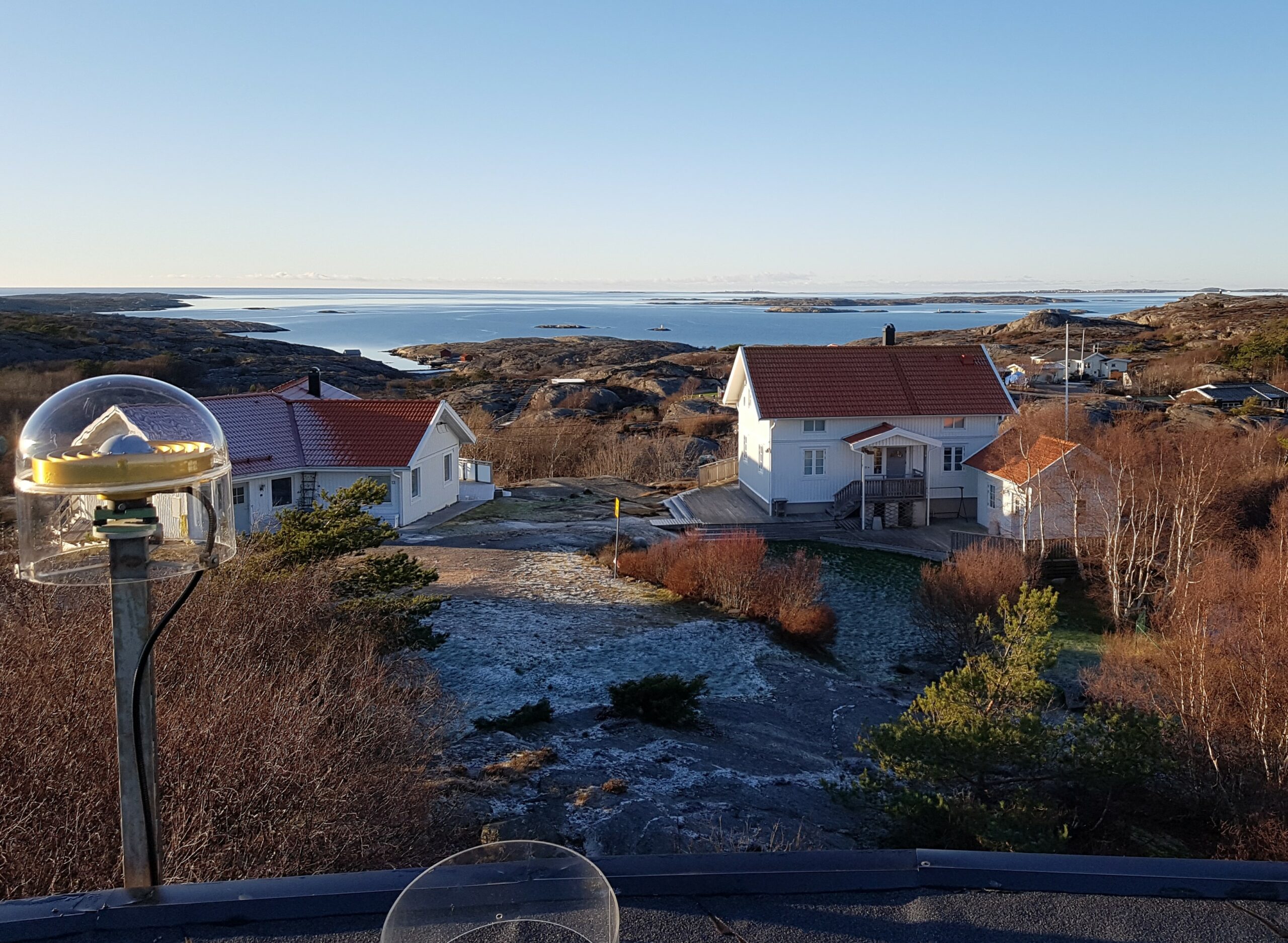
The SWEPOS reference station near the fishing village of Mollösund, in the outer archipelago of Sweden’s west coast. Shown is a custom clear radome that SWEPOS designed with extra-long skirt to keep the rain and snow from blowing in and freezing the connections.
The primary mechanism for managing the data from these stations, and for creating the real-time outputs, is commercial RTN software, although investment has been made in substantial customization, for instance in account and invoicing management, and its own online post-processing service. SWEPOS began with GPSNET, from the German GNSS developer Terrasat (now part of Trimble) two decades ago and continues to this day. The software continued to evolve, and is presently Trimble Pivot Platform, one of the most widely implemented RTN suites globally. SWEPOS uses Pivot to help determine station velocity and has a module to apply this to the solutions. In addition to the CORS, monitoring stations are also included in the network to check the quality of GNSS solutions.
SWEPOS provides accounts for internal use; for other users it has a subscription model. One customer has more than 300 subscriptions. For some external commercial corrections service providers, they utilize SWEPOS CORS data and pay a flat rate for the CORS data they purchase. SWEPOS has been exploring how best to provide data for the growing autonomous vehicle markets as well.
In recent years, overall usage has increased dramatically. “We just logged 2,574 simultaneous users,” says Wiklund. “This is an increase of 8 percent compared to the same period last year. A large part of the increase is in the construction industry, where the technology is available on machines so that you can position yourself and measure accurately when digging.
“Another area of increase is in agriculture. Among other things, it enables tractors to drive accurately,” continues Wiklund. “When spring farming started early, this was reflected in the usage. In addition to the record number of concurrent users, SWEPOS has never before had so many unique users––around 4,160––in one day.”
From the usage logs in Pivot, operators have determined that construction is the highest sector at about 47 percent. “And we have 12 percent municipal surveyors, who were the biggest users when we first started this service,” says Wiklund. “Now we see surveyors at about 19 percent of total usage, and agriculture at 9 percent.”
To give an idea of the value of an RTN, we would need to contrast the time/costs of doing similar tasks with legacy methods. A value proposition circulated among many RTN in the early days of implementation in various countries, based on testing and feedback from early adopters. While the savings per hour would vary greatly for different applications, a rough number of $100 per hour for surveying applications like project control is considered. If that was applied to 2,500 concurrent users, the value would be staggering. But often usage is seasonal for some applications, limited to weekdays for others, and many users may only connect for an hour or two each day. Even if the multiplier were halved to take into consideration non-survey applications, 1,000 connections for a few hours per day could still add up to millions saved annually.
Reliability
For its many users and collaborative applications, consistent availability is a high priority for SWEPOS. It has redundant hardware and software set up offsite from the primary center, with a failover mechanism so that the CORS can stream to the secondary system if the primary is not available, and corrections can be accessed from the backup caster.
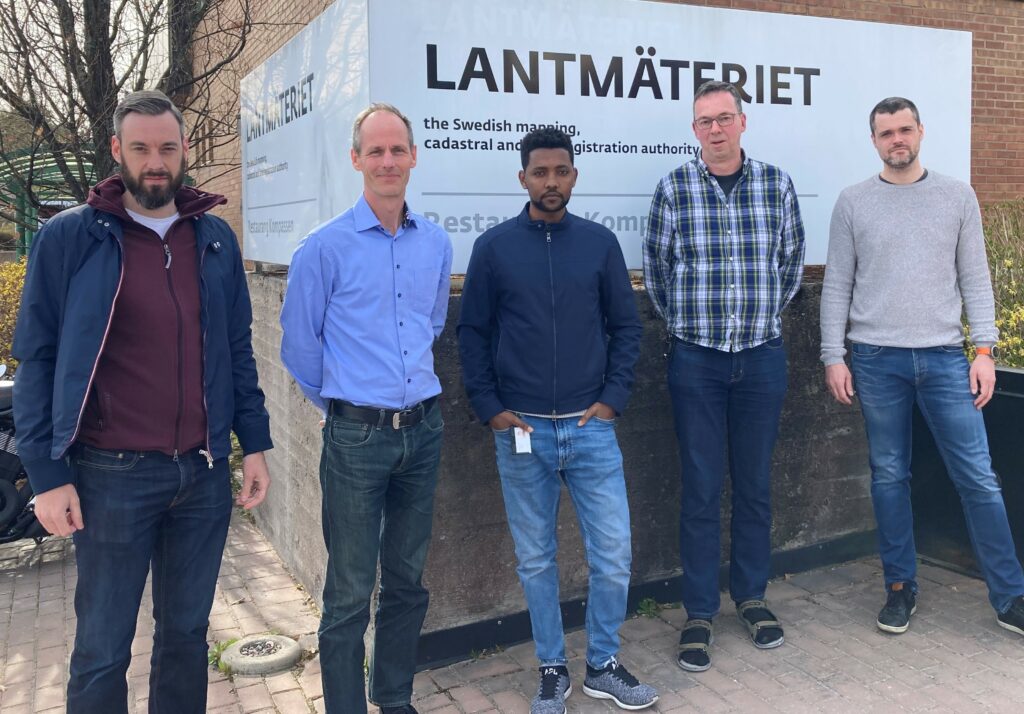
Part of the SWEPOS team, from left to right: Martin Sundlöf, GNSS development; Peter Wiklund, head of SWEPOS; Kibrom Ebuy Abraha, GNSS research and development; Anders Frisk, GNSS development; Martin Håkansson, GNSS research and development.
“From this high availability (HA) system, we had only approximately 30 minutes of downtime for all of last year,” says Wiklund. “Some new applications, such as autonomous cars, can’t have any downtime at all. This was actually a reason for implementing the HA solution.”
There is also a disaster recovery configuration in place in cooperation with the National Land Survey. There are clones of all critical servers that can be spun up at a moment’s notice as needed. An SMS messaging system also keeps users apprised of system status.
R&D
While Lantmäteriet provides many other services, SWEPOS is well staffed, including research and development specialists. They have scientists and specialists in geodesy, information technology, and GNSS engineering.
Its land database preceded the use of GPS, so operators developed their own user interfaces and customized the relevant RTN elements to suit. Similarly, the system had been using geodesy software (e.g., Bernese) prior to and during the expansion of the foundational CORS network. And while Pivot has tools to do this, SWEPOS continues to use its own system to constrain the network to the national geodetic reference framework.
As a real-time network operator, SWEPOS is a leader in research that can benefit network operators worldwide. An example is the publication of the 2021 paper: “GNSS SWEPOS Data Quality Monitoring – Signal Disturbances Detection System” [ bit.ly/3MHCWSn ], co-authored by Wiklund and Lantmäteriet colleagues Dr. Kibrom Ebuy Abraha, Anders Frisk, and Mats Westberg. The core research was done in conjunction with the Swedish Defense Research Agency. The paper outlines the development of an SNR signal-to-noise-ratio-based interference detection system that has been added to the daily monitoring operations. Whether the interference is inadvertent, deliberate, or hardware related, the potential has grown with the adoption of multiple constellations; more signals mean more to monitor.
The vibrant R&D activities of Lantmäteriet has attracted world class researchers and engineers to their operations. For example, there is Dr. Abraha, GNSS developer at Lantmäteriet, who previously served as a Survey, Alignment and Metrology Engineer at SLAC National Accelerator Laboratory at Stanford. There is also Dr. Martin Håkansson, GNSS researcher at Lantmäteriet, who outlined another key research project that will leverage SWEPOS infrastructure and data to aid an ambitious mapping project for the Baltic Sea.
“The project is to map the Baltic Sea in greater detail because it was never mapped as well as it should have been,” says Håkansson. “The maritime sector is really looking for this. For example, a chart might show where someone identified a rock, and it might show one elevation, but it might have been the elevation of the sea floor. Was it 123 meters? Was it eight meters? With the uncertainty, should we avoid this route?”
The solution they are developing would utilize GNSS, improved gravity models, and depth determinations. “If you have a good map of the bottom of the Baltic Sea, together with a good theory model, you can determine accurate keel clearances,” adds Håkansson. “They can save quite a lot of money by optimizing the routes and knowing where they have enough water on the keel to go over shallow water.”
The challenges of using GNSS corrections from a real-time network over broad stretches of the sea are multi-fold, but Lantmäteriet is working steadily to overcome these. There are long baselines without a CORS, so they are looking at seaborne platforms for project CORS. And there are bandwidth limitations of the maritime radio system that will be used to deliver corrections.
“This limitation can be overcome in different ways,” says Håkansson. “Removing some of the constellations would be easiest. But we have also looked into removing specific satellites and optimizing satellite geometry of the many satellites. We’re also considering what correction messages could be omitted to keep the packet sizes small.”
The SWEPOS team has done a lot of work to design CORS that can provide robust performance in harsh environments like the arctic north. They’ve gone as far as designing a unique radome for their GNSS antennas and having it manufactured. It is a clear plastic dome that has an extra-long skirt to keep the rain and snow from blowing in and freezing the connections.
Real-time GNSS corrections data from an RTN is also often utilized for meteorology. SWEPOS provides various atmospheric details that the observed signals can indicate, like hourly perceptible water readings, to the Swedish Meteorological and Hydrological Institute to help improve weather forecasts.
SWEPOS is not alone among RTN’s in providing data to lateral uses in addition to geodetic and GNSS research, it has excelled in many of these activities. Being at the confluence of geodesy, science, and land administration has helped Lantmäteriet pursue many such projects.
I’ve visited the nerve center of several RTNs and interacted with many more operators during my 20 years operating one. But by virtue of the many services it provides, the sheer scale of the operation, education, and experience of the staff, and especially the testing, research, and development done, SWEPOS is “check-all-of-boxes” cool. I’d say it’s one of, if not the coolest RTN.
SIDEBAR
What is and RTN?
Uncorrected, autonomous GPS (or more properly “GNSS” when using multiple satellite constellations) is not very precise; one-meter to 10-meters or more. Various techniques have been developed to yield higher precision-like differential base-rover real-time kinematics (RTK). This is a type of differential computation using a “base” on a known point and creating “correctors” to transmit (typically by radio) to a “rover” seeking to establish positions on unknown points.
Differential RTK was developed and productized in the mid 1990s. One disadvantage of RTK, this single-baseline method, is that the solution degrades the farther away the rover is from the base. In general circumstances, baselines of more than 10 km might yield inconsistent results on different days. Other disadvantages include the need to license radios, extra equipment costs, and time needed to set up a base.
Various entities like cities, states, utilities, private firms, and whole countries recognized the value of differential base-rover RTK and how much could be saved by using this new technology compared to conventional surveying and mapping techniques. Networks of single-base RTK stations began to pop up, but with a 10-km spacing for optimal results, it would be impractical to cover large areas with bases and radios.
But the late 1990s saw two technological advances: cellular networks so that corrections could be transmitted by Internet protocols, and Network RTK. Known as NTRK, or more commonly as RTN, these real-time networks that deliver corrections via cellular systems made broad coverage practical. There was a tremendous boom in RTN development from 2000 to 2010. A popular type of RTN solution is virtual reference station (VRS), developed by Terrasat (now part of Trimble) in the late 1990s (https://bit.ly/3PnjhJO), the solution used by SWEPOS and hundreds of other real-time networks.
Another key development was an Internet data protocol from the German geospatial agency BKG, called NTRIP (network transport of RTCM via Internet protocol). This international, non-proprietary protocol has been integrated into the surveying field software of nearly all manufacturers of high-precision GNSS equipment. Most RTN provide non-proprietary correction types, like RTCM3.2-MSM, that can be used by nearly any modern GNSS rovers. RTN’s serve many applications: surveying, mapping, geodesy, precision agriculture, construction, public safety, precision navigation, atmospheric sciences, and other geosciences like plate tectonics studies and earthquake early warning systems. Many users also rely on RTN/CORS data for post-processing. RTN in infrastructure has become a vital component of, if not the default infrastructure for geodetic reference frameworks.
Globally, there are many excellent RTN of all types and sizes. Public, private, cooperative, vendor corrections services on a continental scale, and city RTN consisting of five CORS to arrays of 1,200 like in Japan. There is the pioneering national network in Switzerland, SWIPOS; the organization of individual state RTN in Germany called SAPOS; the OSNET operated by the Ordnance Survey of the UK, and many more.
The global community of RTN operators are often in communication with each other, sharing ideas, tips, and solutions, and even coordinating international conferences. It is the nature of RTN that if they’re not operated well, reduced quality is obvious to end users. So, in a way, RTN is its own
“Canary in a Coal Mine:” it either works well or not at all.
RTN are fairly ubiquitous in many parts of the world, quietly providing the precise “where” that a geospatially demanding world often takes for granted. Visions of “metaverses” will only be realized with a lot of 3D data capture, and that requires a lot of precise positioning—RTN will be right there.
There are excellent complimentary services, like real-time precise point positioning (PPP). For example, Trimble CenterPoint®️ RTX,™️ and new PPP/RTN hybrids are being developed for growing markets like vehicular autonomy. Whatever the future of high-precision positioning technology holds, the infrastructure that RTN provides will continue to be a vital amenity.