New applications for smallsat constellations are growing from autonomous formation flying.
Space Flight Laboratory (SFL) of Toronto is on a mission to expand the utility of small satellites in a variety of commercial, government, and research sectors. Among its current focus areas is exploit- ing its successful heritage in autonomous formation flying, a technology that enables multiple satellites to work together and perform functions not typically possible with just one.
Above: A HawkEye 360 uses small satellites in autonomous formation flight to develop geospatial solutions based on radio frequency survey conducted from space.
SFL is reputed to be the only organization to have accomplished precise, autonomous formation flight on two nanosatellites, CanX-4 and CanX-5, with centimeter-level knowledge and sub-meter level control accuracy in low-Earth orbit. The space-proven, compact technology is available at a low cost, enabling commercial exploitation not previously possible.
“The most significant benefit of this technology is in commercial geospatial services,” said Dr. Robert E. Zee, director of SFL. “Certain remote sensing and geolocation applications become viable when two, three, or more satellites are flown in precise orbital configurations.”
Autonomous formation flying means the satellites are orbiting in a constellation with their relative positions predetermined and spacing precisely maintained without assistance from ground-based commands. Autonomy refers to the fact that onboard hardware and software allow the satellites to communicate with each other to keep their positioning exact.
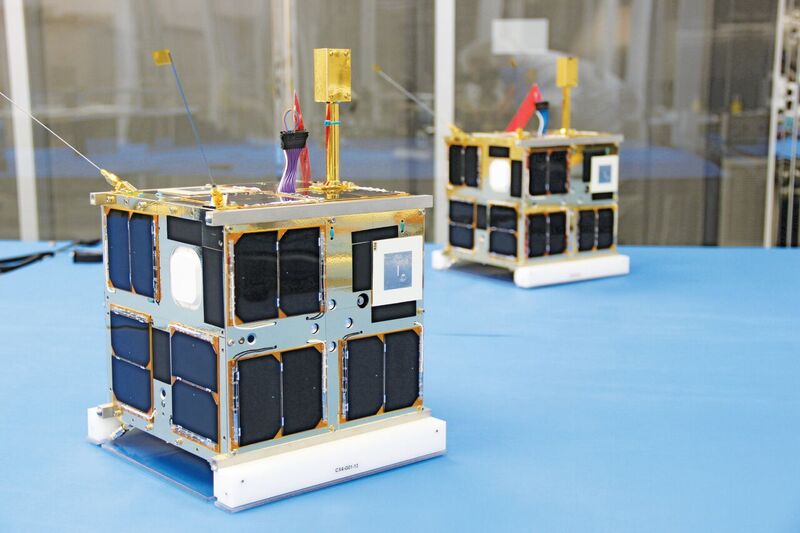
CanX-4 and CanX-5 in an SFL clean room prior to a successful 2014 mission. Credit: Space Flight Laboratory.
“For space-borne sensing and localization missions, autonomy is the key because the real-time position determination and control continuously preserves the precise configuration, which establishes a more accurate positional baseline to process the measurements and data being collected,” said Zee.
Not all satellite constellations use, or need, autonomous formation flying, he added. For example, clusters of small Earth-observation satellites now being launched—to collect large volumes of imagery on frequent revisit cycles—don’t necessarily require the technology.
SFL, on the other hand, is focused on enabling a different set of applications that would not be possible, or would be prohibitively expensive, with single satellites or uncontrolled constellations.
Proof of Concept Completed
SFL was established at the University of Toronto Institute for Aerospace Studies as a self-sustaining specialty lab servicing the world. It builds smaller satellites on tight schedules and at low cost.
In the past two decades, the organization has developed 25 nano-, micro- and small satellite missions that have totaled more than 68 years of orbit time. They have spanned the full spectrum of space-borne applications including Earth observation, environmental sensing, and communications.
Over the course of these diverse missions, the SFL has innovated precise attitude control, modular power systems, and numerous subsystems designed specifically for smaller satellites, which have contributed to the development of autonomous formation flying capabilities.
Others who have accomplished formation flight to date have done so with larger, more expensive satellites, or with much less accuracy, which makes commercial implementation and utility virtually impossible. Formation-flying capabilities exist for traditional satellites, but operational deployment in many applications, especially related to commercial geospatial and location-based services, has been impractical due to the cost of building multiple large satellites. These other technologies do not scale well to smaller satellites because of both the size and expense of the components and subsystems involved.
SFL, however, achieved a microspace breakthrough with the development of the CanX- 4 and CanX-5 demonstration mission in 2014. Funded by SFL and the Canadian government, the two 7kg nanosatellites flew autonomously in five planned orbital configurations with separations varying from 50 meters to one kilometer. Their positional accuracy was less than a meter, and relative position knowledge was within a few centimeters.
“We believe SFL is the first to demonstrate autonomous formation flying of nanosatellites at a cost that is feasible for commercial operation,” said Zee. “And the innovations we developed to make this possible scale up from nanosateillites to microsatellites and small satellites. It’s easier to make something big if it is small, but much harder to make something big smaller.” While CanX-4 and CanX-5 are nanosatellites, or under 10 kg, bigger satellites such as microsatellites (10-100 kg) and small satellites (100-500 kg) are also direct beneficiaries of the SFL technology.
Practical Benefits
Geolocation services
Using autonomous flying formation for geolocation services involves three or more satellites receiving radio frequency (RF) signals from transmission sources on the ground (either active or passive) and using triangulation to precisely calculate their locations in three dimensions.
“The accuracy of the location calculation depends on how precisely the separation of the satellites is known and controlled,” said Zee.
One of the first companies to create a commercial business around satellite-based geolocation is HawkEye 360 in Herndon, Virginia. Their development team, led by Deep Space Industries, selected SFL to build a demonstration constellation comprised of three 15-kg microsatellites set for launch in early 2018.
HawkEye 360 is creating an RF survey-based geospatial information network that will map and analyze RF signals coming from numerous communications and transportation sources, including air, sea, and land vehicles. Among its applications will be identifying wireless broadcast interference sources for communications companies and expediting the location of emergency beacons in search-and-rescue operations.
“Automated formation-flying makes our mission both possible and differentiated,” says Chris DeMay, founder and COO of Hawk-Eye 360.
Sparse aperture sensing
In this application, a cluster of satellites equipped with small sensors capture data that is then combined to provide the effect of a single instrument with a larger aperture than the individual satellites in the cluster can independently carry.
SAR images from different satellites in formation flight can be processed and combined for higher resolution imagery.
For example, synthetic aperture radar (SAR) images from different satellites in formation flight can be processed and combined for higher resolution imagery. This enables the use of smaller antennas and smaller satellites.
Other SAR applications include interferometric SAR (InSAR), where an interferogram of two SAR images is made from SAR images captured by formation flying satellites to provide real-time change detection.
“As with triangulation, the accuracy of combining these multiple data sets into meaningful information relies on knowing the precise baselines, or relative positions, of the sensors on the satellites,” said Zee.
Clusters of electrooptical sensors might leverage formation flight to identify and track movement of objects on the ground or to capture overlapping image pairs for use in stereo photogrammetry.
Software and Hardware Integration
The autonomous formation flying technology developed by SFL is a complex choreography of hardware systems and software algorithms. They work together to keep the satellites in their intended orbital configurations with predefined baseline separation among them.
SFL devised closely coupled sets of algorithms for attitude control, navigation, and formation control of the satellites. These software solutions interact primarily with three onboard hardware systems: propulsion, carrier phase differential GPS, and S-band inter-satellite radios.
Navigation is provided by the GPS receivers, which SFL has adapted from terrestrial units to minimize cost and size. The data from the GPS units are then analyzed to determine the relative phases of reception among the formation flying satellites that, in turn, determine relative separation distances. The GPS hardware and navigation algorithms determine relative position to within a few centimeters on orbit.
With this information, the on-board formation control algorithms then actuate satellite propulsion systems to counteract orbital perturbations and control relative position in the chosen orbital configuration to sub-meter accuracy.
“The satellites receive sig- nals from the same GPS satellite but at slightly different times,” said Zee. “The phase difference between the signals is key to determining the baseline separation between the satellites.”
CanX-4 and CanX-5 were particularly challenging given their small 20x20x20cm size. For the individual satellites to receive signals from the same GPS satellites, they had to mirror each other in orientation to point their GPS antennas in the same direction.
SFL developed an extremely precise attitude control system for its nanosatellite buses, taking into account the fact that thrusters can only be placed on one of the exterior surfaces of each satellite due to its small size. Thrust commands were coupled with the attitude and formation control systems to maintain orientation and position.
“In operational formation flying missions, more thrusters and more GPS antennas would be used in order to enable the attitude control system to point instruments while in formation,” said Zee, “In many ways, the CanX-4 and CanX-5 mission was so challenging that the success has opened the door to much easier formation flying missions that would be implemented in practice.”
SFL has developed several different propulsion system technologies to maneuver satellites in orbit. For the CanX- 4/-5 mission, cold gas was used for the sake of simplicity, but SFL has developed warm gas, monopropulsion, and cylindrical Hall thruster systems for small satellite missions requiring higher efficiencies and longer durations in orbit.
Critical for the control algorithms to operate in a coordinated fashion is the inter-satellite communications link. The CanX-4 and CanX-5 satellites carried onboard S-band radios that shared data between them, most importantly their attitude and GPS location information.
“The control algorithms on the CanX-4 and CanX-5 satellites are identical, giving each the ability to assume the role of chief or deputy,” said Zee. “The deputy controls its position relative to the chief. In the case of more than two satellites, there would be multiple deputies all controlling their positions relative to the chief.
“Having identical satellites allows the roles of the satellites to be swapped if necessary to provide for maximum flexibility, redundancy, and lifetime. This illustrates the benefits of forma- tion flight which include satellite redundancy and graceful degradation.”
For CanX-4/CanX-5, onboard radios receive commands from the SFL ground station in Toronto to move the satellites into specific configurations, but once there the satellites maintain formations on their own.
Most operational formation missions are likely to use only one particular configuration depending on the mission applications, but the formations can change in flight.
What’s Next
SFL believes autonomous formation flying will become a mainstay capability for the majority of small satellite constellations in the near future, primarily because the affordability of the technology now makes many multi-satellite missions financially viable that simply were not so in the past, especially in the commercial sector.
“Small satellite constellations, in general, are the future for geospatial and numerous other application areas,” added Zee.
“Small satellite constellations, in general, are the future for geospatial and numerous other application areas.”
There is strength in numbers. Individual satellites in a constellation can fail or be replaced, often without mission degradation, and the constellation itself can be upgraded incrementally over time with new additions. Large clusters of multiple satellites are also difficult to jam from the ground, and hence provide electronic protection against denial of service threats.
“By following a true microspace approach that leverages miniaturization, modern component performance, and low cost without sacrificing quality, formation flying technology is now available for worldwide commercial applications,” said Zee. “We are excited about the future possibilities.”
To read the rest of the articles in this print issue, click on the issue cover, below.